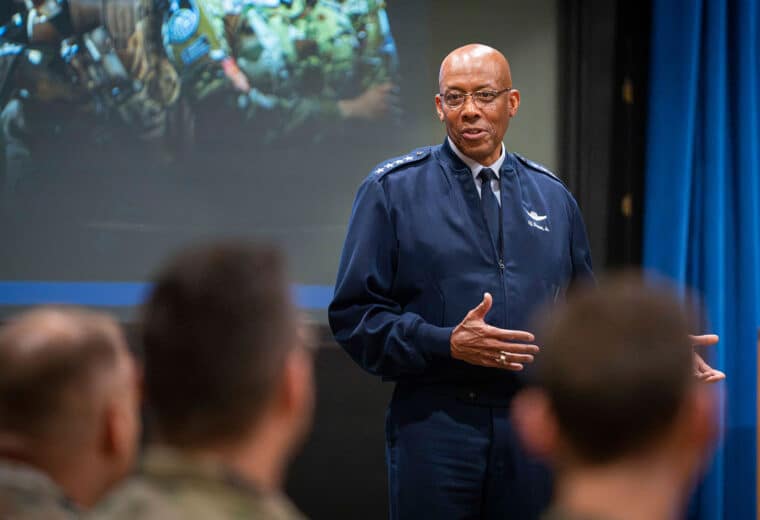
Gen. Charles Q. Brown Jr. to be Executive-in-Residence at Duke
Former Chairman of the Joint Chiefs of Staff will engage with students and faculty through Duke Engineering’s Character Forward program.
We’re sorry—the news story you were looking for has been archived.
Please see the most recent stories below.
Former Chairman of the Joint Chiefs of Staff will engage with students and faculty through Duke Engineering’s Character Forward program.
Gunsch brings a broad range of leadership experience in engineering, operations and strategic consulting to the MEM program.
With a career spanning both academia and industry, Dr. Bozic brings a unique blend of scholarly expertise and practical business insight to her new role.